-
Stay on schedule
Anticipating flight test requirements keeps projects on critical path. By planning parallel subsystem build-up tests, surprises are minimized later in the development cycle. The process is smooth, and smooth is quick.
-
Control the amount and types of risks
Flight test risk management does not mean zero risk, but includes powerful tools to anticipate specific hazards and identify mitigations.
-
Avoid costly redesign
Flight test reinforces requirements traceability with tools like system engineering. By incorporating “testability”, product teams can minimize shifting goalposts and anticipate interfaces across the design.
China’s mysterious space plane returns to Earth after 9-month
The robotic vehicle stayed aloft for 276 days on its second orbital flight.
The second orbital mission of China’s robotic space plane has come to a close. The mysterious reusable vehicle touched (May 8, 2023)down at the Jiuquan Satellite Launch Center in northwest China, wrapping up a 276-day mission to Earth orbit. The success of the experiment marks an important breakthrough in China’s research on reusable spacecraft technologies, which will provide more convenient and affordable round-trip methods for peaceful use of space.
The space plane launched from Jiuquan on Aug. 4, 2022, kicking off a mission short on details but long on intrigue. On Oct. 31, the vehicle ejected something into orbit, possibly a service module or a small satellite designed to monitor the Chinese space plane. Western experts think the vehicle is roughly similar to the U.S. Space Force’s robotic X-37B, which is about 29 feet (8.8 meters) long. The U.S. military is similarly tight-lipped about the X-37B, which has flown six orbital missions to date, the longest of which lasted 909 days.
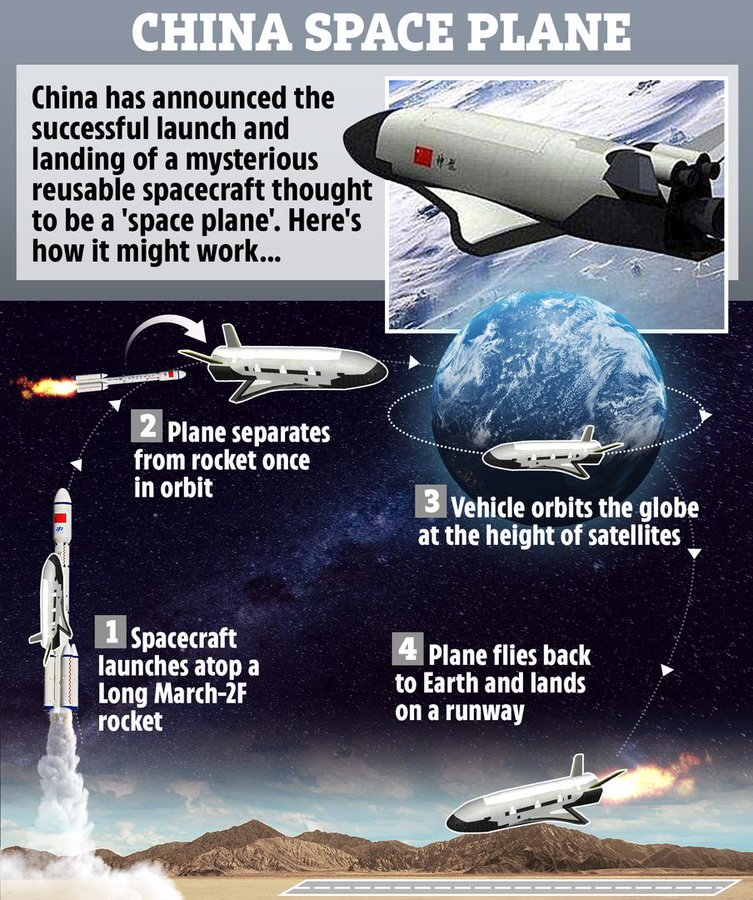
Other reusable spacecraft or spaceplane projects are under consideration in China. The China Aerospace Science and Industry Corp. (CASIC) is working on its own spaceplane, named Tengyun, while commercial firm Space Transportation last year raised more than $46.3 million for its hypersonic spaceplane plans.
A number of Chinese rocket companies have also created presentations including small spaceplanes launching atop concepts for liquid rockets.
NASA tests rotary detonation engine: it will revolutionize space travel
Rotating detonation rocket engine for deep space exploration
Propulsion technology represents a key element for the realization of advanced space missions, both as regards the exploration of the Moon and Mars, and as regards the transport of cargo and people to destinations even further away in deep space. To reach this goal, engineers studying new forms of propulsion at NASA developed and tested the first rotary detonation engine, or also known as the RDRE.
The operation of a rotary detonation engine is based on supersonic combustion, known as detonation, which allows it to generate more thrust using less fuel than currently used propulsion systems. The detonations and supersonic waves that are generated inside a combustion chamber are very complex to manage, and therefore the project acquires even more importance given the state of progress.
This technology has been talked about since the 1950s, but to be honest, when it was theorized it seemed more utopian than real, at least until today. This type of engine could be used to power both human landers and interplanetary vehicles for the most distant destinations in space, such as the Moon and Mars, and could represent an alternative to other technologies currently under study, such as the nuclear thermal one of which we have talked about recently.
NASA Marshall Space Flight Center engineers and lead contributor IN Space LLC conducted a test firing on the RDRE in 2022, confirming the data obtained. The RDRE achieved its main goal by demonstrating that its equipment, made with 3D printing techniques, could operate for extended periods while withstanding the extreme conditions generated by detonations. During testing, the RDRE produced approximately 2 tons of thrust for approximately one minute with an average chamber pressure of 47 kg per square inch, the highest pressure level recorded for this type of engine.
The RDRE uses GRCop-42, a copper alloy developed by NASA, and the powder bed fusion process to allow the engine to operate in extreme conditions for longer periods.
The results obtained during the tests represent an important step towards the use of this technology on future spacecraft, which will allow NASA and the commercial space industry in general, to carry ever-increasing payloads to destinations in deep space, a fundamental element to make space exploration more sustainable.
Following the positive results obtained with the RDRE, NASA engineers are working to develop an even more powerful fully reusable RDRE engine, to further identify the performance advantages over traditional liquid engines. We leave you with the video of the incredible rotary detonation engine test.
How Rotating detonation engine works ?
A rotating detonation engine (RDE) is an engine using a form of pressure gain combustion, where one or more detonations continuously travel around an annular channel. Computational simulations and experimental results have shown that the RDE has potential in transport and other applications.
In detonative combustion, the results expand at supersonic speed. It is theoretically more efficient than conventional deflagrative combustion by as much as 25%. Such an efficiency gain would provide major fuel savings.
Disadvantages include instability and noise.
The basic concept of an RDE is a detonation wave that travels around a circular channel (annulus). Fuel and oxidizer are injected into the channel, normally through small holes or slits. A detonation is initiated in the fuel/oxidizer mixture by some form of igniter. After the engine is started, the detonations are self-sustaining. One detonation ignites the fuel/oxidizer mixture, which releases the energy necessary to sustain the detonation. The combustion products expand out of the channel and are pushed out of the channel by the incoming fuel and oxidizer. Although the RDE’s design is similar to the pulse detonation engine (PDE), the RDE is superior because the waves cycle around the chamber, while the PDE requires the chambers to be purged after each pulse.
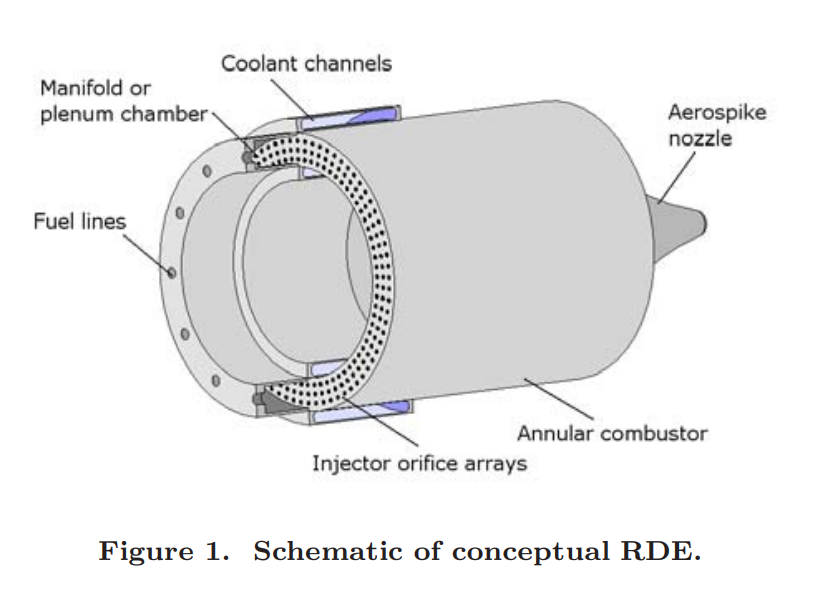
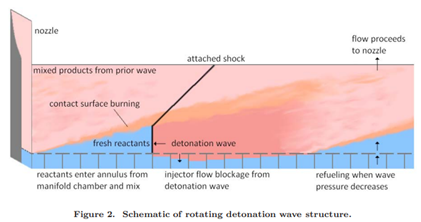
A possible RDE configuration is shown schematically in Fig. 1 while an unwrapped schematic of the rotating detonation wave is shown in Fig. 2. Reactants are fed either separately or premixed into an annular combustor from the bottom. A detonation wave and possibly multiple waves rotate in the annulus just at the exit of the injector arrays, consuming the reactants feeding continuously from the bottom. The high pressure is then reduced to the inlet pressure after passage of the detonation wave which allows the reactants to again feed into the annulus, thereby allowing chemical reactions to continue to sustain the detonation wave. The reactants penetrate a certain distance into the annulus which roughly marks the end of the detonation wave. Further away, the detonation wave degenerates into a blast wave. This structure has previously been called a combined detonation-shock wave35,36 or detonation wavelet. The figure shows a postulated contact surface burning due to the hot environment exceeding the autoignition temperature. Figure 2 shows penetration of the hot, high pressure products just downstream of the detonation wave into the injector. A complex wave interaction is set up, dependent on the properties of the reactants and products, as well as on the geometry of the injector. Preliminary indications are that such a scheme can be operated safely.
Just 45 Days to Get to Mars by nuclear propulsion, NASA and DARPA will test it soon
Nuclear Propulsion Could Help Get Humans to Mars Faster
NASA and DARPA are to demonstrate a nuclear thermal rocket engine in space, with a view to using the technology to send crewed missions to Mars. The Demonstration Rocket for Agile Cislunar Operations (DRACO) program will develop and flight test in space nuclear thermal propulsion technology that could be used to transport astronauts to Mars and beyond. The research agencies plan to send the DRACO test vehicle into space and run the nuclear engine in 2027.
NASA’s Space Technology Mission Directorate (STMD) will lead technical development of the nuclear thermal engine, which will be integrated with an experimental spacecraft developed by the Defense Advanced Research Projects Agency (DARPA). A nuclear thermal rocket engine uses a fission reactor to generate extremely high temperatures. The engine transfers the heat produced by the reactor to a liquid propellant, which is expanded and exhausted through a nozzle to propel the spacecraft.
Engineers believe that nuclear thermal rockets can be three or more times more efficient than conventional chemical propulsion. Using a nuclear thermal rocket will enable faster transit times, reducing risk for astronauts. Reducing transit time is a key component for human missions to Mars, as longer trips require more supplies and more robust systems. Other benefits to space travel include increased science payload capacity and higher power for instrumentation and communication, said NASA.
DARPA is acting as the contracting authority for the development of the entire stage and the engine, which includes the reactor. DARPA will also lead the overall program including rocket systems integration and procurement, approvals, scheduling, and security, cover safety and liability, and ensure overall assembly and integration of the engine with the spacecraft. Over the course of the development, NASA and DARPA will collaborate on assembly of the engine.
Nuclear thermal rocket engine testing was last conducted by the USA more than 50 years ago under NASA’s Nuclear Engine for Rocket Vehicle Application and Rover projects. NASA and the Soviet space program spent decades researching nuclear propulsion during the Space Race. A few years ago, NASA reignited its nuclear program for the purpose of developing bimodal nuclear propulsion – a two-part system consisting of an NTP and NEP element – that could enable transits to Mars in 100 days.
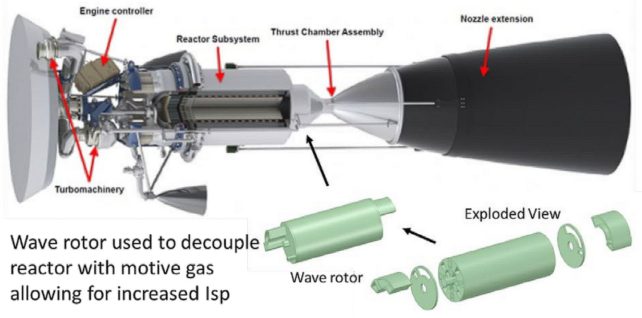
As part of the NASA Innovative Advanced Concepts (NIAC) program for 2023, NASA selected a nuclear concept for Phase I development. This new class of bimodal nuclear propulsion system uses a “wave rotor topping cycle” and could reduce transit times to Mars to just 45 days. The proposal, titled “Bimodal NTP/NEP with a Wave Rotor Topping Cycle,” was put forward by Prof. Ryan Gosse, the Hypersonics Program Area Lead at the University of Florida and a member of the Florida Applied Research in Engineering (FLARE) team.
Gosse’s proposal is one of 14 selected by the NAIC this year for Phase I development, which includes a US$12,500 grant to assist in maturing the technology and methods involved. Other proposals included innovative sensors, instruments, manufacturing techniques, power systems, and more.
For Nuclear-Thermal Propulsion (NTP), the cycle consists of a nuclear reactor heating liquid hydrogen (LH2) propellant, turning it into ionized hydrogen gas (plasma) that is then channeled through nozzles to generate thrust. Several attempts have been made to build a test this propulsion system, including Project Rover, a collaborative effort between the US Air Force and the Atomic Energy Commission (AEC) that launched in 1955.
In 1959, NASA took over from the USAF, and the program entered a new phase dedicated to spaceflight applications. This eventually led to the Nuclear Engine for Rocket Vehicle Application (NERVA), a solid-core nuclear reactor that was successfully tested. With the closing of the Apollo Era in 1973, the program’s funding was drastically reduced, leading to its cancellation before any flight tests could be conducted. Meanwhile, the Soviets developed their own NTP concept (RD-0410) between 1965 and 1980 and conducted a single ground test before the program’s cancellation.
Nuclear-Electric Propulsion (NEP), on the other hand, relies on a nuclear reactor to provide electricity to a Hall-Effect thruster (ion engine), which generates an electromagnetic field that ionizes and accelerates an inert gas (like xenon) to create thrust. Attempts to develop this technology include NASA’s Nuclear Systems Initiative (NSI) Project Prometheus (2003 to 2005). Both systems have considerable advantages over conventional chemical propulsion, including a higher specific impulse (Isp) rating, fuel efficiency, and virtually unlimited energy density. While NEP concepts are distinguished for providing more than 10,000 seconds of Isp, meaning they can maintain thrust for close to three hours, the thrust level is quite low compared to conventional rockets and NTP. The need for an electric power source, says Gosse, also raises the issue of heat rejection in space – where thermal energy conversion is 30-40 percent under ideal circumstances.
And while NTP NERVA designs are the preferred method for crewed missions to Mars and beyond, this method also has issues providing adequate initial and final mass fractions for high delta-v missions. This is why proposals that include both propulsion methods (bimodal) are favored, as they would combine the advantages of both. Gosse’s proposal calls for a bimodal design based on a solid core NERVA reactor that would provide a specific impulse (Isp) of 900 seconds, twice the current performance of chemical rockets.
Gosse proposed cycle also includes a pressure wave supercharger – or Wave Rotor (WR) – a technology used in internal combustion engines that harnesses the pressure waves produced by reactions to compress intake air. When paired with an NTP engine, the WR would use pressure created by the reactor’s heating of the LH2 fuel to compress the reaction mass further. As Gosse promises, this will deliver thrust levels comparable to that of a NERVA-class NTP concept but with an Isp of 1400-2000 seconds. When paired with a NEP cycle, said Gosse, thrust levels are enhanced even further: “Coupled with an NEP cycle, the duty cycle Isp can further be increased (1,800-4,000 seconds) with minimal addition of dry mass. This bimodal design enables the fast transit for manned missions (45 days to Mars) and revolutionizes the deep space exploration of our Solar System.”
Based on conventional propulsion technology, a crewed mission to Mars could last up to three years. These missions would launch every 26 months when Earth and Mars are at their closest (aka. a Mars opposition) and would spend a minimum of six to nine months in transit. A transit of 45 days (six and a half weeks) would reduce the overall mission time to months instead of years. This would significantly reduce the major risks associated with missions to Mars, including radiation exposure, the time spent in microgravity, and related health concerns. In addition to propulsion, there are proposals for new reactor designs that would provide a steady power supply for long-duration surface missions where solar and wind power are not always available. Examples include NASA’s Kilopower Reactor Using Sterling Technology (KRUSTY) and the hybrid fission/fusion reactor selected for Phase I development by NASA’s NAIC 2023 selection.
NASA, the Department of Energy (DOE) and industry are also developing advanced space nuclear technologies for multiple initiatives to harness power for space exploration. Through NASA’s Fission Surface Power project, DOE awarded three commercial design efforts to develop nuclear power plant concepts that could be used on the surface of the Moon and, later, Mars.
Orbital Debris Removal Technologies
Access to space is becoming easier and currently one can launch small spacecraft into Low Earth Orbit (LEO) for a few hundreds of thousands of dollars. According to the U.S. Space Surveillance, there are half a million space objects in Low Earth Orbit (LEO), and only 30,000 are actively tracked. 23,000 pieces of orbital debris are larger than 10 cm and provide more velocity when in orbit. The estimated population of particles between 1-10 cm in diameter is approximately 500,000. There are 100,00,000 pieces of debris that are smaller than 1mm. The United States is responsible for 30% of all orbital space debris. The debris is frequently in transit the orbits of hundreds of operational spacecrafts. Additionally, most of the debris were inserted into long-duration orbits, with orbital lifetimes imagined at multiple decades and centuries. Collisions can lead to huge investment efforts, including expensive fixes. There have been three major collisions since 2005. They all involve a large intact body, typically a satellite, colliding with smaller pieces. With these smaller pieces still in orbit, more collisions will occur, thus creating more space debris. The term “debris removal” implies taking active measures to remove something from orbit and not letting its orbit decay on its own. There are three main removal technology concepts: Laser Solutions, Electrodynamic Tethers, and Deployable Nets.
Active orbit debris removal
De-orbiting of the debris is mainly focused on using different mechanism for changing orbital velocity. This is done by applying a force (or impulse). In the limiting case where the orbital velocity is reduced to zero, the object will only have gravitational potential energy and, thus, “fall” back to Earth. The three technologies considered are different in the way they impart this force or impulse. The lased-based approaches impart this force or impulse in a contactless fashion. Electrodynamic tethers are objects that are deployed from or attached to an object that will result in forces being applied to it form Earth magnetic field. Nets apply this force by physical contact with the object.
As depicted in figure below, all three of the orbital debris removal systems discussed here effectively perturb the original orbit of the object so that it eventually gets captured by the atmosphere.

Laser Orbital Debris Removal (LODR)
Laser orbital debris removal (LODR) is a contactless method in that applying a force or impulse to the object to be de-orbited does not require to physically contact it. The system uses a laser either on Earth or in orbit to shone on the object that is to be deorbited. One of the earliest proposed use of LODR was by NASA in 1996 with Project Orion. Project Orion [1] was created as a “laser broom” to remove all pieces of debris less than 10 cm under 1100 km in two years. This LODR as used a pulsed laser.
The LODR solution is considered successful, especially for larger debris. With having the ability to capture debris from the ground and in space, researchers can explore a solution with multiple options. There are concerns with this laser orbital removal technology capturing the smaller pieces (less than 1mm).
As depicted in the figure below, the laser, which is either a ground- or space-based one applies a force or impulse to the debris. This in turn changes the orbital speed and eventually orbital radius “r”.

The total force imparted to the debris is F and can be resolved into its tangential component T and radial/normal component N. As will be discussed below, the magnitude of T and N depend on many factors including the orientation (attitude) of the debris. The force applied by the laser beam causes a change in the debris orbital velocity which puts it into a transfer orbit with a perigee deep in the dense part of the atmosphere ash shown in Figure 1.
The laser deposits energy into the material of the debris which causes ablation of the material. The ablation products leave the debris at a high speed and carry with them some of the momentum of the debris. In effect, the ablation products are acting as a miniature thruster that is applying an impulse to the debris. The net thrust that is imparted by the ablation products depends on the orientation of the debris. As show shown in Figure above, if the flat part on which the laser shines is not perpendicular to the original velocity vector, the resulting force will have both a tangential component T and a normal component N.
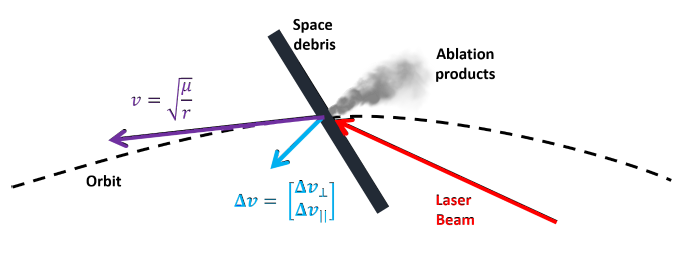
Referring to Figure 3 above, the magnitude of the tangential velocity change |Δv| applied to the debris is described by the following equation:
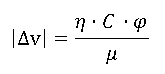
The efficiency factor ƞ accounts for the combined effects of improper thrust direction on the target, target shape and tumbling. The term φ is the laser fluence which is a measure how much energy is contained in the laser. The mechanical coupling term C measures how much of how effectively the laser energy is converted into momentum change of the debris. The last term in this equation μ is the debris areal mass density and is a description of the debris’ geometry.
The equation above shows, the change in orbital velocity achieved by LODR depends on many factors including the laser being used as well as the geometry and construction of the debris’ host spacecraft.
Below are reported the characteristics of LODR on common materials used on spacecraft ([2], [3]).
Debris Material | Laser Fluence Required (J/m2) | Mechanical Coupling Coefficient |
Aluminum (Al) | 11.7 | 3.94E+29 |
Gold (Au) | 16.9 | 2.20E+36 |
Carbon (C) | 15.68 | 3.20E+27 |
Iron (Fe) | 13 | 1.19E+32 |
Lithium (Li) | 10.4 | 1.05E+23 |
Molybdenum (Mo) | 5.2 | 5.46E+36 |
Tungsten (W) | 20.8 | 2.48E+38 |
Below are reported the Advantages and Disadvantages of LODR
Advantages:
- Contactless, no interaction with space debris
- lasers can work with most materials commonly used (see tab above)
Disadvantages:
- Difficulties to be aware on the orientation of the debris (required for tangential velocity change)
Electrodynamic Tethers (EDT)
Electrodynamic Tethers (EDT) are systems that take advantage of Earth’s magnetic field to generate an electromotive force which can be used apply a positive or negative Δv to an object in orbit. Tethers are long strands of fibers that are used to connect or couple objects together to operate as one system. In space, tethers are typically launched or directed into Earth’s orbit and will align with the movement of Earth’s magnetic field ([4], [5], [6], [7]). Some tethers will convert potential energy to kinetic energy while others act as motors. Thus, they can be used as a deorbiting system or a “boost” system for changing the orbit of Earth orbiting objects. In other words, some tethers will convert potential energy to kinetic energy while others act as motors. This solution has been predicted better for mega-constellations due to the amount of electrodynamic drag they possess. There have been several EDT demonstrations missions most the TSS-1 and TSS-1R mission, Plasma Motor Generator (PMG) experiments and NASA’s Propulsive Small Expendable Deployer System or ProSEDS mission.
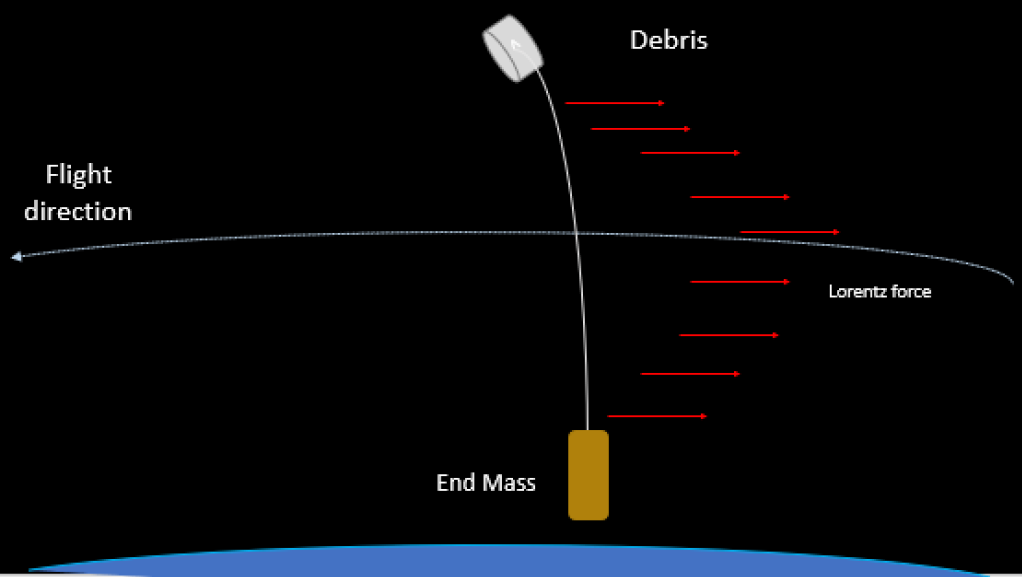
Figure 4 above shows the principle of an electrodynamic tether solution for removing orbital debris. The tether was deployed and is shown orbiting around the Earth with an end mass. Current runs through the tether, working along with the geomagnetic field of the planet. The bare side of the tether attaches itself to the orbital debris using a hooker or connecter. After capture, the end mass allows the system to rotate and release the tether and orbital debris. The system then deorbit itself and burns up into the atmosphere, removing the debris overall. This concept is used in the KITE experiment from JAXA. Previous studies show that debris objects that should be removed from crowded orbits can re-enter the Earth’s atmosphere within one year. Having a system with at least a 10-km tether on a host satellite should be able to eliminate that [8].
If a space craft can deploy a long cable of length L cable of carrying an electric current I, then as it orbits the interaction between Earth’s magnetic field B and the cable will generate a force given by:

Figure below shows a simplistic diagram of the relationship between the orientation of the cable and the force F that would be generated by a deployed cable in orbit. The result force will be orthogonal to both the cable and Earth’s magnetic field. As such, it acts in the direction opposite to the velocity vector of the object that deployed the cable. Thus, it is effectively delivering negative Δv to the space craft and causing its orbit to decay.
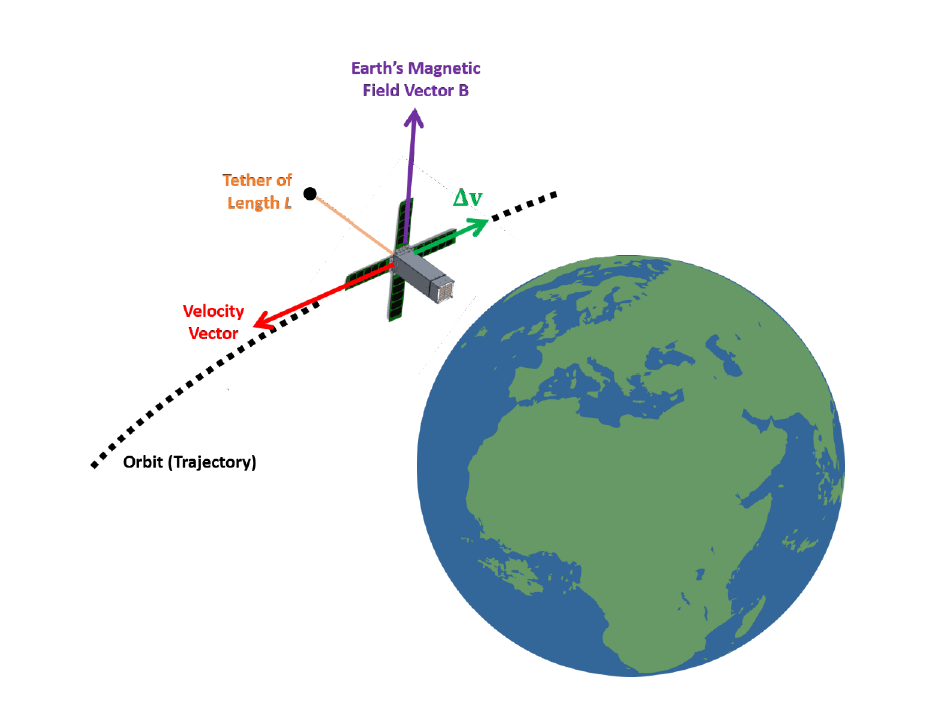
Below are reported the Advantages and Disadvantages of Electrodynamic Tether:
Advantages:
- Being EDT simple and passive device, it can be easily installed on many small satellites
- It can be used not only to de-orbit a small spacecraft but also for increasing their orbital altitude as well.
- it can be used to generate electrical power which can be used to charge batteries thereby supplementing the function of solar panels. Unlike solar panels, however, they can generate power regardless if the sun is in view or not
Disadvantages:
- Risk of impact with orbital debris
- It only works on objects that have it designed and built into them. This means that it cannot be used on random space debris unless there is a way to attach a compact and self-contained EDT system to the debris on orbit.
- Mechanism used for reeling out the cable can be difficult to design, they can be made to be rather compact and fit in most small space vehicles
Deployable Nets
Deployable nets are perhaps the easiest to understand technology that has been proposed for orbital debris removal. The idea is to deploy something akin to a fisher’s net called a tether-net from a chaser spacecraft (still connected to the net) in the proximity of orbital debris. The tether-net is deployed ahead of the debris to be removed. The debris slowly drifts into and gets tangled by the tether net. Then the chaser spacecraft fires thrusters to change the orbit of itself and of the debris in the net such that they will reenter Earth’s atmosphere and burn.
While conceptually simple, the design of the nets themselves and the debris removal operation using them can be complex. For example, proposed nets are a collection of elastic rods connected. The rods can be connected by knots, or elastic joints. As a deformable body, the elastic rod can bend and form around the target to secure and capture it. Most nets considered for orbital debris removal are Cosserat nets. Cosserat nets consist of a network of elastic rods and elastic joints that link together to create a deformable body [9].
Furthermore, nets need to have a closing mechanism to allow satellites to release net once debris is captured. These can also be complex to design and operate in space.
The deployable net solution can be explained using the practices of fishing. Fishermen locate the fish and eject a net from a safe distance. The net comes with a closing mechanism to secure capture and reel the net back up to the surface.
Figure 5 below shows the similar concept occurring with the deployable net solution in space. The net is transported into space on a host satellite in a container. Once the satellite is at desired altitude, the net is deployed and captures targeted debris using its closing mechanism. The closing mechanism would be the end points on the corners of the net to ensure proper capture. The satellite will then travel to a lower altitude and cut the net with the attached debris for it to de-orbit and burn up in the atmosphere.

Below are reported the Advantages and Disadvantages of Deployable Nets:
Advantages:
- Unlike the other solutions, deployable nets can capture a large group of debris at one time.
- Due to previous advantage, it is oneof the more cost-efficient solutions.
Disadvantages:
- The Net needs proper spatial awareness for proper capture. Without proper attitude and control practices from the host satellite, this would be difficult for the net to capture the debris.
- Risk of possible stuck onto the host satellite. This can cause issues in its operation, thus failing the mission
References:
[1] J. Campell, “Project Orion: Orbital Debris Removal Using Ground Based Sensors and Lasers,” National Aeronautics and Space Administration, MSFC, Alabama, 1996.
[2] L. Zhou, X.-Y. Li, W.-J. Zhu, J.-X. Wang and C.-J. Tang, “The effects of pulse duration on ablation ressure driven by laser radiation,” Journal of Applied Physics, vol. 117, no. 12, 31 March 2015.
[3] K.-C. Lee, T. Taira, G. Mo Koo, J. Young Lee and J. J. Yoh, “Ignition characteristics of laser-ablated aluminum at shock pressures up to 2 GPa,” Journal of Applied Physics, vol. 115, no. 1, 3 January 2014.
[4] M. Sandoval, “Space Tethers,” University of Colorado, Boulder, 2007.
[5] M. Dobrowolny and N. Stone, “A technical overview of TSS-1: The first Tethered-Satellite system mission,” Il Nuovo Cimento, vol. C, no. 17, pp. 1-12, 1994.
[6] “Plasma turbulence enhanced current collection: Results from the plasma motor generator electrodynamic tether flight,” Journal of Geophysical Research: Space Physics, vol. 100, no. A2, 1 February 1995.
[7] J. Ballance and L. Johnson, “Propulsive Small Expendable Deployer System (ProSEDS),” AIP Conference Proceedings, vol. 552, no. 1, 4 April 2001.
[8] The Aerospace Corporation, “Danger: Orbital Debris,” 4 May 2018.
[9] J. Spillmann and M. Teschner, “Cosserat Nets,” IEEE Transactions on Visualization and Computer Graphics, vol. 15, no. 2, pp. 325-338, March 2009.
Archer reveals production design for eVTOL aircraft
Archer Aviation unveiled the design of its production aircraft named Midnight at an open house event in California, USA last week.
Midnight is an evolution of Archer’s demonstrator eVTOL aircraft Maker, which can be seen in the video above before the reveal of Midnight. Maker made its first flight in December last year.
The company said the flight test program is “on schedule” and expects to complete a full transition flight in the coming weeks.
The five-seater Midnight eVTOL aircraft uses a proprietary twelve-tilt-six propeller configuration and has been developed for short distance trips of around 20 miles (32km) with a charging time of approximately 10 minutes in-between.
Archer plans to certify Midnight with the FAA during “late 2024” and then operate it within an urban air mobility (UAM) network it plans to launch in 2025.
Adam Goldstein, Archer’s Founder and CEO said, “From day one Archer’s strategy has always been about finding the most efficient path to commercializing eVTOL aircraft.
“We believe our strategy and team’s ability to execute on it has allowed us to establish our leadership position in the market and is why we are confident we will be the first company to certify an eVTOL aircraft in the US with the FAA.”
Archer completed Midnight’s preliminary design review in August and began working towards Type Certification.
Transition from vertical to horizontal flight is a key milestone in any eVTOL aircraft’s development program because it demonstrates a number of key systems, such as power and flight control and validates the flight physics of the design.
The company said that Maker’s flight test program had generated invaluable data that has been used in the development and certification path of Midnight.
“We continue the push towards commercialization, with the vast majority of our resources focused on completing the development and certification of Midnight, building out our manufacturing and supply chain capabilities and hardening our go-to-market plans,” said Mark Mesler, Archer’s CFO.



rfgtsdf
Deal extended: Courses Up To 85% OffFirst Liquid Hydrogen Fuel System Demonstrator for aircraft successfully tested by GKN
GKN Aerospace has successfully ground tested a liquid hydrogen fuel system for aircraft.
The demonstrator was designed, built and tested by GKN and UK-based Filton Systems Engineering to investigate the feasibility of using a liquid hydrogen fuel source in a search and rescue drone.
The system, which is able to vaporize and condition liquid hydrogen for use in the fuel cell, integrated the tank with a distribution solution.
The performance of the fuel system was verified by coupling it with a proton exchange membrane (PEM) fuel cell stack, representative of the type that could be installed on a future zero-emission aircraft.
During testing successful storage and management of the liquid hydrogen was achieved, and the fuel cell power system was supplied with hydrogen at the required temperature and pressure over a range of electrical loads typical of a drone’s search and rescue mission.
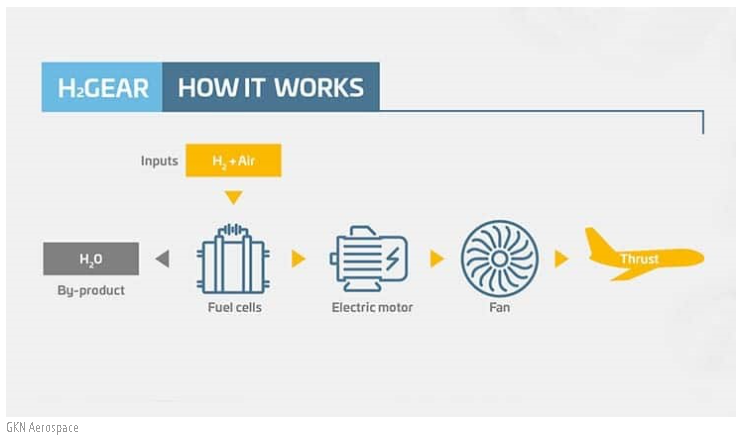
Key outcomes of the project include the development of safe system design, manufacturing knowledge, operational knowledge for liquid hydrogen fuel systems, hydrogen fuel system test data, and an adaptable test rig suited to further study of hydrogen components and subsystems, said GKN.
The project enabled GKN to understand and address many of the safety concerns raised by the introduction of such a novel fuel.
Max Brown, vice president of technology at GKN Aerospace said, ’’We are delighted with the outcome of this project and believe we are very much at the forefront of exploring the challenges in this area.
“In a single end-end test environment the team has demonstrated fueling and storage of liquid hydrogen, conditioning and distribution of cryogenic gas, and the use of PEM fuel cells to generate electrical power.
”While the focus of this work was on a small scale platform, the achievement is highly aligned with other work we are conducting in programs such as H2GEAR, where we are delivering propulsion technologies focussed on enabling zero emissions flight.’’
The fuel system was developed and tested as part of a UK Government funded project called Safe Flight which aims to increase the endurance of unmanned search and rescue drones.
Kratos delivers Valkyrie unmanned drone to US Air Force
Kratos’ Valkyrie XQ-58 drone is a stealthy unmanned combat aerial vehicle designed and built for the US Air Force
Kratos has supplied the first of two XQ‑58A Valkyrie unmanned drone to the US Air Force for use in testing autonomous systems.
The Valkyrie is a low-cost, high-performance unmanned aircraft. It is rocket-launched from a rail system and controlled from a ground station or airborne fighter. An onboard computer system is capable of determining the best flight path and throttle settings to comply with commands.
The Autonomous Aircraft Experimentation (AAx) team is using the 30ft long Valykrie at the Eglin Air Force Base Range in Florida, USA for developmental ground and flight testing.
This is the first time the Valykrie has been used by the military, so much of the aircraft’s infrastructure and logistics have to be created from the ground up and will be recorded.
Maj. John Nygard, 40th FLTS AAx team lead said, “When you combine the XQ-58A with the Eglin Range infrastructure, you get an uncrewed aircraft that enables real, open-air test of flight autonomy software capabilities while also proving out the resource requirements that could be used for future combat collaborative aircraft.”
The AAx team are testing autonomous aircraft in partnership with the Air Force Research Laboratory (AFRL).
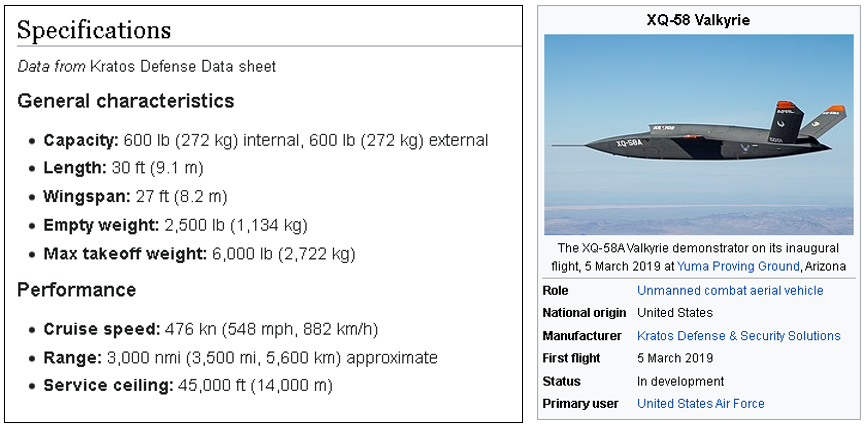

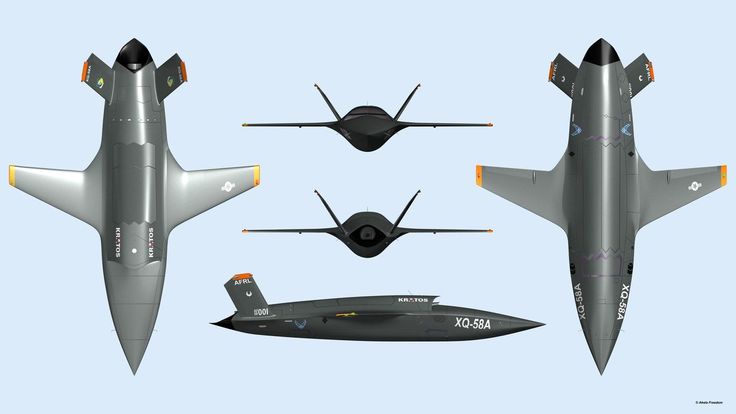
“The data generated during previous tests, along with feedback provided from our user community, show that in order to rapidly develop and mature tactical autonomy on an appropriate timeline, investment in and utilization of appropriate military range resources is required,” said Matthew Niemiec, AFRL autonomous aircraft experimentation portfolio lead.
Military range resources are a major reason Valykrie testing is being conducted at Eglin. The Eglin Range communications supports infrastructure that will allow engineers at the ground station in its Central Control Facility to monitor the vehicle’s performance during flight.
Additionally, autonomous aircraft operations, airspace, and safety processes have been developed to enable safe and effective flight testing for uncrewed air vehicles.
The Valykrie’s first flight is scheduled for later this month.
Nygard described this new test as time-critical, with a lot to get done in a short amount of time. “The goal by fall 2023 is to leverage this platform for experimentation with crewed-uncrewed teaming display solutions,” said Nygard.
Additionally, the AAx team is building a data-storage and simulation environment to capture operator feedback and integrate their inputs into the autonomy software development process. The XQ-58A could host a variety of flight autonomy software solutions that were first tested in the simulator, including those from the Skyborg Autonomy Control System and others provided by third-party government and industry partners.
Niemiec said AFRL is working with multiple industry partners to integrate leading-edge autonomy capabilities onto the XQ-58A.
“The simulation investment enables us to focus flight test efforts of XQ-58A on developing the process to deliver autonomy software capable of accomplishing operator-defined missions to the warfighter,” he said.
Development and design
A XQ-58 Valkyrie deploys an Altius-600 unmanned aircraft system
The XQ-58 Valkyrie falls within the USAF Research Laboratory’s Low Cost Attritable Aircraft Technology (LCAAT) portfolio, whose objectives include designing and building unmanned combat aerial vehicles (UCAVs) faster by developing better design tools and maturing and leveraging commercial manufacturing processes to reduce build time and cost. The role of the LCAAT is to escort the F-22 or F-35 during combat missions, and to be able to deploy weapons or surveillance systems.
The XQ-58 is designed to act as a “loyal wingman” that is controlled by a parent aircraft to accomplish tasks such as scouting, defensive fire, or absorbing enemy fire if attacked. It features stealth technology with a trapezoidal fuselage with a chined edge, V-tail, and an S-shaped air intake. The XQ-58 is capable of being deployed as part of a swarm of drones, with or without direct pilot control. Though the XQ-58 is capable of conventional take-offs and landings, it can also be launched from “nondescript launch modules”, such as support ships, shipping containers, and semi-trailer trucks. Kratos could produce between 250-500 Valkyries per year.
Operational history
The XQ-58’s first flight took place on 5 March 2019, approximately two-and-a-half years from contract award. A total of five test flights were planned in two phases to evaluate system functionality, aerodynamic performance, and launch and recovery systems.
On 23 July 2020, the Air Force awarded contracts to Kratos, Boeing, Northrop Grumman, and General Atomics authorizing the companies to compete for the Skyborg program, an effort to field an unmanned wingman cheap enough to sustain losses in combat but capable of supporting manned fighters in hostile environments; Kratos may use the XQ-58 as its submission, although it was developed separately under the LCASD program and another airframe might be submitted. Kratos was further down-selected, along with Boeing and General Atomics, on 7 December 2020. Submissions were delivered by May 2021 for flight tests in July 2021.
On 26 March 2021, the XQ-58A completed its sixth test flight, opening the doors of its internal weapons bay for the first time and releasing a 27 lb (12 kg) Area-I Altius-600 small unmanned aircraft system (UAS)
What is Artemis? Everything you need to know about NASA’s new moon mission
NASA is embarking on a yearslong, multistage, groundbreaking mission to the moon. Here’s why NASA is returning to the moon, who’s going, what technology is enabling the mission, and more.
Artemis I launch update
After a handful of scrubbed launches, Artemis I finally launched into space on Nov. 16 at 1:47 AM EST. The Artemis I mission will last 25 days before the Orion spacecraft returns to Earth and splashes down off the California coast.
NASA had to scrub its first Artemis I launch attempt on Aug. 29, after encountering a problem with one of the Space Launch System (SLS) rocket’s engines. The engine was having trouble reaching the proper temperature range for liftoff. The second launch attempt occurred on Sept. 3. and was unsuccessful due to a hydrogen leak in the quick disconnect, an interface between the liquid hydrogen fuel feed line and the SLS.
NASA officials have said that scrutiny of its program is warranted — they are spending public dollars, after all. However, they’ve said they will not succumb to pressure to rush the launch.
What is the Artemis mission?
NASA is embarking on a yearslong mission called Artemis that involves a multistage plan to send astronauts to the moon and beyond. The Artemis mission will culminate with landing the first woman and person of color on the moon.
This mission will prepare humanity for the long journey to Mars, and it will help us establish a sustainable lunar economy. NASA is working with international and commercial partners to carry out the mission.
Why is it called Artemis?
From 1969 through 1972, the NASA Apollo program took humans to the moon. Artemis is the twin sister of Apollo and the goddess of the moon in Greek mythology.
The Artemis I mission patch (figure below) showcases the Space Launch System (SLS) rocket carrying the Orion spacecraft. The triangular shape is a classic shape for NASA mission emblems dating back to the shuttle era. It represents the three main programs that constitute NASA’s Deep Space Exploration Systems: Orion, SLS, and Exploration Ground Systems. Several elements within the design carry symbolic meaning for this historic flight.

Why are we going back to the moon now?
NASA and its partners have made huge strides since the Apollo program, as demonstrated by the success of the International Space Station. Humans have continuously lived and worked aboard the ISS for two decades. The ISS, however, is just 250 miles above Earth. The moon, by contrast, is 250,000 miles away, while Mars is 140 million miles away.
If humans want to establish a longer-term presence beyond low Earth orbit (where the ISS is located), returning to the moon is the next logical step.
“There are many reasons to go back, or as you may have heard me say, go forward to the moon,” then-NASA Administrator Jim Bridenstine wrote in 2019. “With Artemis, we’re going to explore more of the moon than ever before, and we’re planning to stay this time. We are traveling 250,000 miles to the moon to demonstrate new technologies, capabilities and business approaches needed for future exploration of Mars, which can be as far as 250 million miles away from home.”
What are the goals of the Artemis mission?
In the broadest terms, the goals of Artemis are to enable scientific discovery, open up new economic opportunities, and inspire a new generation of scientists, technologists, and leaders.
By returning to the moon, NASA aims to find water and other resources that will support long-term space exploration. Along the way, the agency expects to learn more about the moon, Earth and the universe. Ultimately, establishing a presence on the moon will give NASA and its partners the knowledge and operational confidence necessary to make it to Mars.
Meanwhile, NASA’s mission should create new economic opportunities on Earth and beyond. There’s already a momentum behind a nascent space economy that, according to NASA leaders, could in 20 years take public and private missions beyond low Earth orbit. NASA aims to stimulate services and infrastructure development on the lunar surface and in cislunar space.
The space economy is already a $400 billion industry “and on the way to $1 trillion, and I suspect it’ll get there faster than we think,” said James Reuter, associate administrator for the Space Technology Mission Directorate (STMD) at NASA, earlier this year.
As for inspiring the next generation, there’s definitely evidence that space exploration inspires young people to study science. This goal also explains why NASA is committed to sending the first woman and the first person of color to the moon.
“Our job at NASA is to do the things that are difficult, and to do the things that are right, and to motivate our base, which is our youth,” NASA’s chief astronaut, Reid Wiseman, recently said. “And right now, our country is a diverse and extremely rich country… We want every kid in America to look at our poster and say, ‘Oh, I see myself in that… I can do that someday.'”
What is the mission timeline?
Should the Artemis I mission launch on Nov. 14, it would result in a mission duration of about 25-and-a-half days with a splashdown in the Pacific Ocean on Friday, December 9.
Back in early August, NASA said it aims to launch the Artemis II mission in 2024. That mission will send astronauts on a lunar flyby test, making it the first crewed mission to go beyond low-Earth orbit since 1972.
Then, in 2025, NASA aims to launch the Artemis III mission, sending the first woman and the first person of color to the surface of the moon.
However, there’s a good chance the mission will fall behind schedule. Preparing for such a huge mission just takes time. For instance, Axiom and Collins Aerospace — the two companies building the next-generation spacesuits that NASA will use in the Artemis mission — said they expect to be able to demonstrate the suits around 2025. Given those types of constraints, NASA Inspector General Paul Martin told Congress in early 2022 that the Artemis III mission “likely will slip to 2026 at the earliest.”
The timeline has been a point of contention since the Artemis mission’s beginning. When then-President Donald Trump in 2017 called on NASA to return to the moon, the agency initially expected to make it back by 2028. In 2019, the Trump administration established a more aggressive timeline, aiming to bring astronauts back to the moon by 2024. NASA has since said the Artemis III mission will launch in 2025 at the earliest.
What is the goal of the Artemis I mission?
The Artemis I mission is unmanned. It will serve as a test of NASA’s deep space exploration systems, making sure the agency is ready to send astronauts to the moon and beyond.
The mission has three main objectives. Its primary goal is to demonstrate that the Orion spacecraft’s heat shield can withstand the high speed and high heat it will experience at lunar re-entry conditions. When Orion returns from the moon, it will be traveling about 24,500 miles an hour. The spacecraft will experience temperatures half as hot as the sun outside the heat shield.
Artemis I’s second objective is to demonstrate the operations and flight modes of the rocket and the spacecraft — all of the facilities across all of the mission phases. During the flight test, teams will verify the launch vehicle and spacecraft systems, such as communications, propulsion, and navigation systems. As part of this objective, NASA is looking for further confidence that Orion, while carrying humans, can tolerate the extreme thermal environment of deep space.
The third objective is to retrieve Orion after splashdown. While engineers will receive data throughout the course of the mission, retrieving the crew module after splashdown will provide information to inform future flights. There will be three mannequins aboard the spacecraft that will help NASA understand how the vehicle fared.
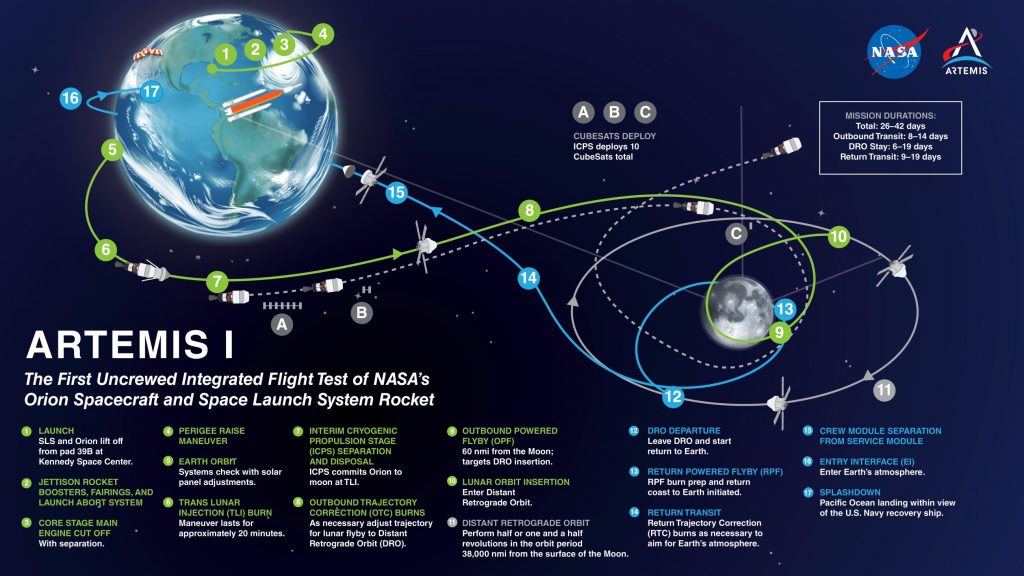
What is the goal of the Artemis II mission?
The Artemis II mission will send four astronauts on a flight around the moon. In just over 10 days, they’ll travel 4,600 miles beyond the far side of the moon. The flight will take the astronauts farther into the solar system than anyone has traveled before.
The point of this mission is to further confirm that NASA’s spacecraft systems are ready to take a crew to deep space.
The crew will be able to test the Orion spacecraft’s life support systems, as well as its communication and navigation systems. Orion will briefly fly beyond the range of GPS satellites and the Tracking and Data Relay Satellites of NASA’s Space Network — this means its crew will rely on the agency’s Deep Space Network to navigate and to communicate with mission control.
Who is NASA sending to the moon?
NASA has yet to decide which astronauts will travel to the moon. The agency has said it hopes to choose which astronauts will fly aboard Artemis II later this year.
The agency will primarily consider technical expertise for all of the Artemis missions, as NASA’s Wiseman recently said. That includes “the ability to dive into literally any situation, any technical need of the vehicle, to understand when things aren’t going quite right, and to understand when they are.”
Beyond that, he said, NASA is looking for team players who can work well with each other and flight directors. Wiseman also stressed the significance of sending a diverse crew to the moon, noting that the incoming class of astronauts represents “all walks of life.”
In the meantime, NASA’s team of 42 astronauts and 10 astronaut candidates are going through rigorous training. It involves landing Army helicopters, studying rocky terrain in areas like Iceland, spending extended periods at the bottom of a pool, and training in VR simulations.
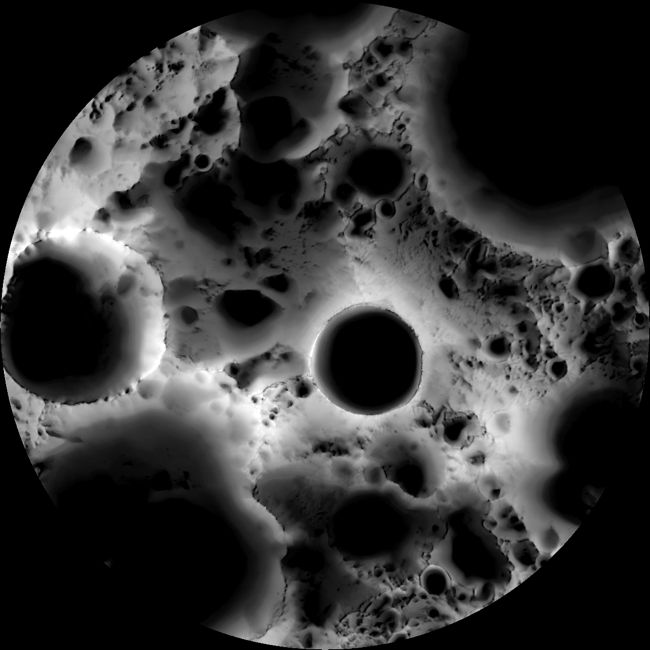
NASA/GSFC/Arizona State University
What will NASA astronauts do on the Artemis III mission?
The Artemis III mission will take astronauts to the lunar south pole, an area of the moon where humans have yet to set foot. Scientists expect the lunar south pole to be rich in potential resources, including water. The astronauts will search for these resources and explore opportunities to make use of them.
The crew will also build an Artemis Base Camp on the moon and work on expanding the Gateway — an outpost that will orbit the moon to provide support for long-term missions on the moon as well as for deep space exploration.
Following Artemis III, NASA intends to launch crewed missions to the moon about once a year.
What spacecraft are being used?
The entire Artemis mission depends on NASA’s Space Launch System (SLS) rocket and the Orion spacecraft. To land astronauts on the moon, NASA will dock Orion at the Gateway. The astronauts will transfer to the Starship human landing system (HLS) that’s being built by the company SpaceX.

SpaceX
Who are NASA’s partners in this mission?
NASA is leading the Artemis missions, but it has several international partners, both from the public and private sectors.
More than a dozen countries have signed the Artemis Accords, which lay out shared principles grounded in safety and transparency for governing space exploration, as well as scientific and commercial activities in space.
Some of NASA’s biggest collaborators are sure to send their own crew members on Artemis missions. Earlier this year, the US committed to including a Japanese astronaut aboard the lunar Gateway outpost. The US also expressed its support for sending a future Japanese astronaut to the moon as part of the Artemis program.
The European Space Agency is also a significant contributor to the Artemis program. The ESA, for instance, designed Orion’s service module — the part of the spacecraft that supplies air, electricity and propulsion. In exchange for the service modules, the ESA will receive three seats on future Artemis missions, Space News reported.
How much will the Artemis mission cost?
n November 2021, the NASA Office of Inspector General (OIG) published an audit of the Artemis program, finding that NASA had already spent around $40 billion on the series of missions. The OIG said it expects the agency to spend around $93 billion by 2025.
As Space.com notes, the US spent $28 billion on NASA’s Apollo program between 1960 and 1973, according to the nonprofit The Planetary Society. That’s about $280 billion in today’s dollars.
Artemis mission: How you can track Orion’s trip to the moon in real time
By using the Artemis Real-time Orbit Website (AROW), anyone with internet access can see where Orion is in space.
The real-time tracker will also show trip information such as mission elapsed time, velocity and spacecraft distance from the Earth and moon.
You can access AROW at any time by simply visiting the website or by following Orion Spacecraft’s Twitter account where you will get updates on travel status. The Twitter account will also provide vectors for Orion’s location that will pinpoint exactly where Orion is located in space. Otherwise visiting the NASA website: https://www.nasa.gov/specials/trackartemis/

Flight Test 101
Flight Test 101: Safe, successful development of high-risk products
This course introduces aerospace professionals to flight test concepts for efficient development of successful products in elevated risk environments. Focus on strategies for test planning, risk management, and team structure in flight test programs.
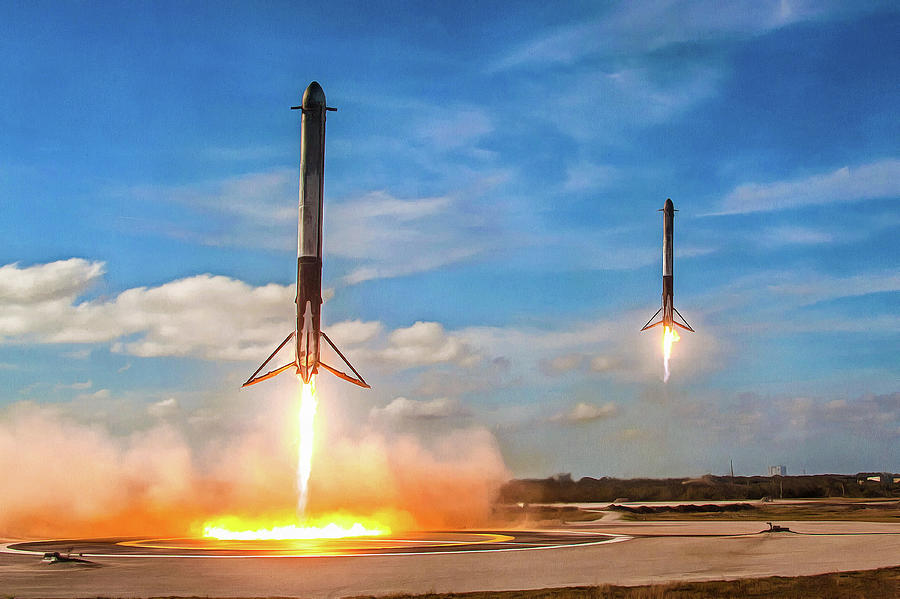
Flight Test for Success – Efficient development, reduce costly failures
Learn the fundamentals of flight test in about 90 minutes
Product teams and businesses using professional flight test methods are over twice as likely to result in a successful product, and complete operational development in one third the time.Any new aerospace product is a bet on the future. Development timelines are usually measured in years, and require a multidisciplinary engineering team. Early application of professional flight test methodologies is proven to substantially reduce development costs and timelines, while improving customer and mission focus.
Yes, you should apply the principles of professional flight test long before there is a product to test.
Flight test practices are relevant across engineering disciplines and enhance both the aerospace professional and product team. This course’s skill sets and tools accelerate product development and avoid costly disasters. Flight test is a team sport.
Who benefits from Flight Test 101?
Every team member contributing to aerospace product development benefits from learning to think like a test pilot or flight test engineer! Whatever your role in building the future of flight, it helps to understand the proven tools for success.
-
Program managers
-
Engineers: Mechanical/Electrical/Software/System/Aerospace/…
-
Artificial Intelligence and Autonomy Researchers
-
Senior engineers
-
Data Scientists
-
View the free preview lesson and see for yourself!
Knowledge is Power
Why is aerospace product development different from making automobiles or consumer electronics?
These characteristics are generally not the case for aerospace.
Value-Added
Flight test principles create value for aerospace development teams
Enroll now, learn at your own pace:
Answers to these questions, and many more! This course is ideal for engineers, program managers, and executives contributing to an aerospace product team. Taught by an experienced test pilot, with content tailored to tech startups and cross-cultural team building.
-
What’s the first flight test that should be flown?
-
How do we balance safety with efficiency?
-
What’s the difference between a databand and tolerance?
-
Who’s in charge during test execution, and what’s the communications plan?
Relevant, Practical, and Applicable
Flight test 101 is applicable to not only to conventional aircraft designs, but also emerging development trends, including:
- Vehicle autonomy
- Remotely / Optionally Piloted Vehicles
- Advanced Air Mobility (AAM) / Urban Air Mobility (UAM)
- Hypersonic flight
- Space launch
- Swarming drones
- Manned/Unmanned Teaming
